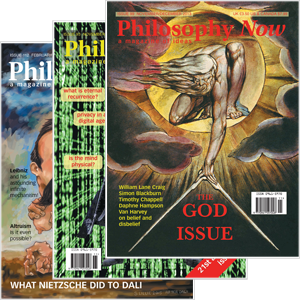
Your complimentary articles
You’ve read one of your four complimentary articles for this month.
You can read four articles free per month. To have complete access to the thousands of philosophy articles on this site, please
Tallis in Wonderland
The Soup and The Scaffolding
Raymond Tallis finds some scaffolding in his soup.
A little while back, the world woke up to the announcement that the team led by the flamboyant American molecular biologist and entrepreneur Craig Venter had created the world’s first artificial life form. A brand new organism, rejoicing in the name ‘Synthia’, had seen the light of day in his lab. He told eyes-on-stalks journalists that this success had changed his “view of the definition of life and of how life works.” The implication was that it should have the same effect on the rest of us.
Many fellow scientists were less excited. They pointed out that Venter had not actually ‘created artificial life’, as the headlines fed by his press releases had proclaimed. Yes, he had synthesised a section of DNA from basic chemicals; but lone DNA is not life. More, much more is needed. In this case, this more was provided by an existing bacterium, whose lowly day job was to cause mastitis in goats. The DNA was inserted into the bacterium. Yes, Synthia was a new life-form, but nearly all of her life had been taken off-the-shelf from nature. Venter had added to the techniques by which scientists can create genetically modified organisms, and this may bring important benefits; but this is a long way from making entirely synthetic life forms, and even further from creating those unhappy creatures of the literary imagination, half-man, half-chemical, stalking the earth, causing havoc, demanding citizenship, and posing insoluble dilemmas for ethicists.
The Atom of Life
Anyone who claims to have created artificial life needs to do more than persuade DNA to hitch a ride in existing cells. But the enormous complexity of living cells makes the task of genuinely synthesising new living matter unimaginably difficult. The decade that has passed since the hysteria surrounding the completion of the Human Genome Project has shown us how little the genetic code tells us about any living organism, and even less about complex ones like you and me. The rise in the Noughties of epigenetics, post-genomics and integrative biology shows that cracking the genetic code has taken us only a small way towards understanding living things. There is a huge gap between the supposed Book of Life, with its complex instructions for making proteins, to cells, never mind whole organs and organisms. The multitude of interacting organelles [ ‘organelle’ means ‘little organ’] within a single cell – the nuclei, mitochondria, the exquisitely folded smart membranes, and their mind-bogglingly complex signalling systems – raise knotty conceptual questions which Venter’s unfounded claim to have created life inadvertently underlines. Of particular interest to me are those questions summarised in the dichotomy between The Soup and The Scaffolding.
The cell, the atom of living tissue, is very small – as little as one billionth of a cubic millimetre. At one level, it may be seen as a sac of chemicals – a soup. Everything within it has to interact with – that is to say, bump into, combine with or break down into – everything else. However, any given cell has numerous discrete functions. At its very simplest, we can divide these functions into those that are concerned with the cell’s survival as a living entity (maintenance and repair); those that are concerned with its replication; and those that are concerned with the job it has to do on behalf of the organism – such as contracting (muscle cells), secreting chemicals (glands), or mopping up invaders (leucocytes). These functions need to be kept distinct: hence the exquisitely complex structure of the cell – the scaffolding. There are obvious cellular structures inside the cell: for example, organelles such as mitochondria, which power other activities in the cell; or the nucleus, which is itself bounded by membranes visible on an electron microscope. And then there are less well-defined structures, such as ribosomal particles, sacs of chemical transmitters transported around the cell, and the endoplasmic reticulum folded throughout the cytoplasm around the nucleus. The challenge of understanding the way cells work and, more broadly, how the anatomy does the biochemistry, and vice versa – how the macro divides into the micro and the macro adds up to the macro so that dinner becomes chemicals and chemicals become flesh and blood – is often disguised by the manner in which living processes are represented on the pages of textbooks and scientific papers by equations or line diagrams that present them as separate paths, not as elements dissolved in a shared bath. Flow charts and pathways make it all very neat. The mystery of life is that it is able to create the most immediate conditions of its own continuation while being anything but neat.
Scaffolding Soup
For a long time, there was controversy as to whether some of the minute cellular structures were real, or whether they were merely artefacts of the staining methods used to clarify intracellular structures. It is not surprising that the allocation of entities either to the soup or to the scaffolding was often disputed. This question touches on something central to the mystery of living tissue: that things need to be kept apart (for the differentiation of function), and yet they have to be brought together (for the integration of function). The scaffolding keeps the complex biochemicals apart, and the soup is where they come together. For example, the mitochondria have the primary responsibility of being the powerhouse of the cell, gathering the energy necessary for many of the other energy-consuming activities associated with life. (Living matter consumes energy even when it is doing nothing except keeping alive, because it is a thermodynamic freak: it is a relatively low entropy/high order phenomenon in a universe whose overall tendency is towards high entropy and zero order.) Its high energy function requires that the mitochondrion should be sealed off from other cellular events. The energy, however, has to be delivered to the places or processes that need it. So the mitochondrion has to be in communication with all the other structures in the cell.
The tension between ‘keeping separate’ and ‘bringing together’ is replicated at every level in an organism, from the integration of the distinct activities and outputs of organs, such as the liver, to the integration in the cell of the distinct activities and outputs of small stretches of the endoplasmic reticulum in the vicinity of, say, a mitochondrion. The cell’s semi-permeable membranes, which hold back some chemicals, allow others through, and actively transport other substances in one direction, may seem to create a compromise between soup and scaffolding, but they do not really solve the problem of integration at every level, so that organelles, cells, organs and organisms act as a whole.
It does not make things less mysterious if we replace the notions of scaffolding and soup with that of structure and function. There are no ‘pure structures’ – no biological containers for life that are a different type of thing to the life that is contained. The containers themselves are living, and that which is contained is also structured. Even the bones of the skull – the most impressive and important of the body’s containers – are living tissue. And structure goes all the way down to individual molecules. The shape and the folding of proteins determines what can and cannot happen in the cell, keeping some things apart and bringing other things together. The interstices of the protein molecules form little nooks, where processes can take place without interference. This is structure at the nanoscopic level.
Reducing Life
The fantastic advances in our understanding of living matter that we have seen over the last few hundred years have depended mainly on teasing organisms apart, and, very often, reducing the scaffolding to soup. The centrifuge, which beats living tissue to a pulp, has been as informative as the dissecting microscope. In short, we have been very successful in identifying individual pathways of biological events and tracing how they interact with one another, and how those interactions are regulated so that the chemical cogs work in synchrony. For example, there is huge knowledge of the enzymes which broker the marriages between molecules and keep unwanted potential suitors at bay. Enzymes speed up certain chemical reactions trillion-fold, so that biologically important processes are promoted, while mere accidents resulting from chemical co-habitation are relatively disadvantaged. For some, this chemical acceleration along with the micro-structure of protein molecules, is sufficient to explain how having all the reagents together doesn’t result in chaos in the nano-lab that is the living cell. (See, for example, Richard Dawkins’ brilliant exposition in The Greatest Show on Earth, 2009.) But as the flow diagrams which describe what happens in cells become increasingly complex, the way in which the cell’s occupants are kept apart at one level and come together at another becomes increasingly mysterious. There is the need, for example, for enzymes to be precisely located, to ensure that they can preside over the bonding of the right molecules. For this reason, it is not enough for the cell to have a stable structure at the molecular level: the enzymes have to travel to the precise places they’re needed – huge distances away when viewed from the molecular standpoint. All of this, of course, needs to be automated. There are no intra-cellular intentions.
What we see at every level of biology is a variant of the problem of ‘the one’ and ‘the many’: in this case the problem takes the form of many distinct processes kept apart by structures (which, being alive, are themselves concatenations of processes), that have to come together to work as a whole – as one. Indeed, we don’t have to look down a microscope or examine the contents of a test-tube to encounter this problem. Consider your own conscious field, with a million experiential elements that are at once separate and also part of the unified ‘I-world-now’ of which you are aware. Or the thousands of ongoing narrative strands, with their background soup of implicit sense, of undeclared but presupposed cognitive frameworks, on which we dance as we construct our sense of ourselves and actively lead our lives. This contrast between the soup and the enabling framework is analogous to that between events and the structure that makes sense of them, or between the breathing that is the uttered word and the syntax that makes it expressive.
It is easy to see why many philosophers, most notably Henri Bergson, resisted the reduction of life to mechanisms mediated by chemistry – to what the great biochemist Frederick Gowland Hopkins characterised as ‘a polyphasic system in dynamic equilibrium’. But the alternatives on offer, whether Bergson’s ‘vital impulse’, or a nebulous ‘life force’, hardly advance our understanding of life. Even so, while we may reject these explanatory gap-fillers, we should at least acknowledge the problem of the integration of the millions of distinct processes which make up living tissue, and be equally sceptical towards those who think that current biological concepts – useful and illuminating though they are – will take us all the way to understanding life. The increasing emphasis on ‘integrative biology’ is a reminder that it is easier to take life apart than to put it together again in order to recover the physiology and anatomy from the chemistry. We should not fall for the hype of Craig Venter’s press machine and believe that we can actually create life.
© Prof. Raymond Tallis 2011
Raymond Tallis’s latest book Michelangelo’s Finger: An Exploration of Everyday Transcendence (Atlantic) is now out in paperback, and Aping Mankind: Neuromania, Darwinitis and the Misrepresentation of Mankind (Acumen) will be published soon.